Can a building skin behave like an animal skin?
Biology scaled architecturally.
Sea creatures like squid, krill, and tilapia adaptively shade through the expansion and contraction of fluid-filled cells underneath their skin. Buildings, on the other hand, adaptively shade through the actuation of mostly rigid electro-mechanical components that tend to be costly, energy-intensive, and prone to failure over time. As buildings account for almost half of the energy footprint of the developed world, the design of climate-adaptive and energy-efficient building skins is vital. Accordingly, we have developed a bio-inspired architectural skin, modelled after pigment-cell activation within sea creatures.
Work physically completed by Raphael Kay and Kevin Nitiema, with remarkable external guidance and mentorship from Charlie Katrycz (UofT, Materials Engineering) and Benjamin Hatton (UofT, Materials Engineering).

Left: Artificial chromatophore expansion for adaptive shading in building skin (Kay et al., 2022)
Right: Biological chromatophore expansion for adaptive shading in Tilapia skin (Tomlinson et al., 2009)

Top: Biological chromatophore expansion for adaptive shading in Tilapia skin (Tomlinson et al., 2009)
Bottom: Artificial chromatophore expansion for adaptive shading in building skin (Kay et al., unpublished, 2020)
Can a window network breathe?
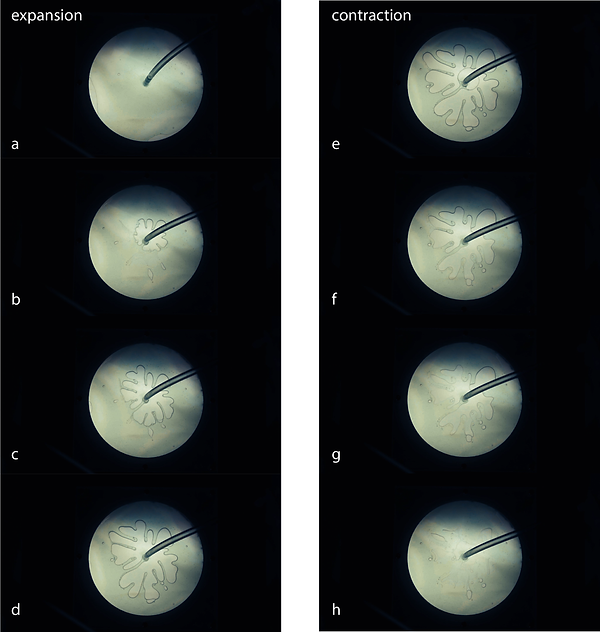
Here, we examine the possibility of a pneumatically actuated expanding and contracting fluidic channel network, operating as a lung. By actively varying the pressure with which a non-viscous fluid (air) is introduced in a viscous fluid, a fluidic network self-forms and self-dissolves, allowing for adaptive thermal and optical capability. More specifically, through pneumatic actuation, we can control the nature (size, geometry) and very existence of a channel network embedded in a window membrane with fluid transportation capability.

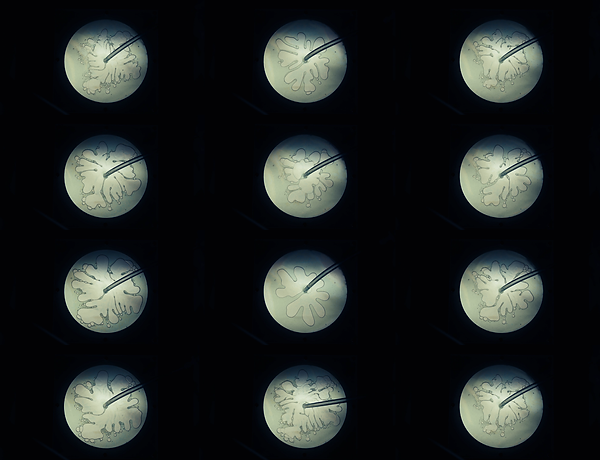
Variation in air pocket geometry as a function of the magnitude of introduced pressure.

Variation in air pocket geometry as a function of the magnitude of introduced pressure with multi-port air introduction.
Breathing, automated.
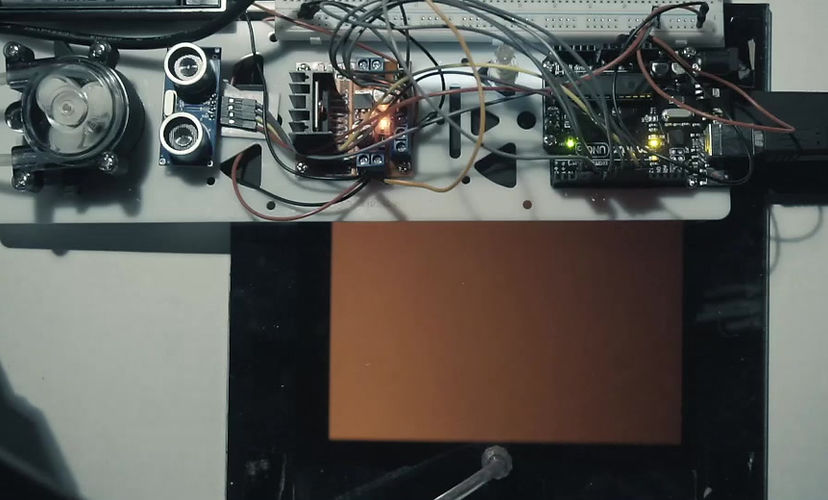
The above figure shows the successful automation of the formation and dissipated of a fluid pocket, in this case by motion input. In the future, we hope that a biological photo-organism will act as an input sensor, as is the case in nature.

The above image shows the reversible self-formation and self-dissipation of a fluid pocket within a more viscous fluid (molasses), all embedded within a rigid window frame.

The figure to the left shows the temperature and light intensity change with the formation of a fluid pocket over a 10 minute long period. The initial temperature was recorded once stable (20 minutes) to be 28 C, and the peak temperature was recorded once stable (3 minutes) to be 37 C. The final temperature was recorded, again once stable, to be 28 C, demonstrating the reversible thermal effect of fluid pocket activation.

The above figure shows iterations of a Diffusion-Limited Aggregation Model. This model approximates the growth of viscous fingering patterns, and can accordingly approximate the geometry of the fluid pockets in question for adaptive shading along building skins. These images were produced using code written by Golan Levin.
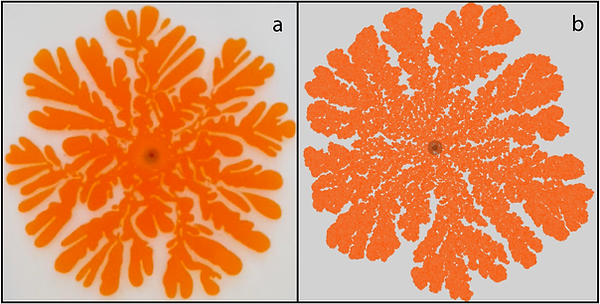
Left: physical prototype of fluid pocket growth. Right: simulation of fluid pocket growth.